New Insights Into How the Nerve Connection Machinery Remodels Itself
Contact
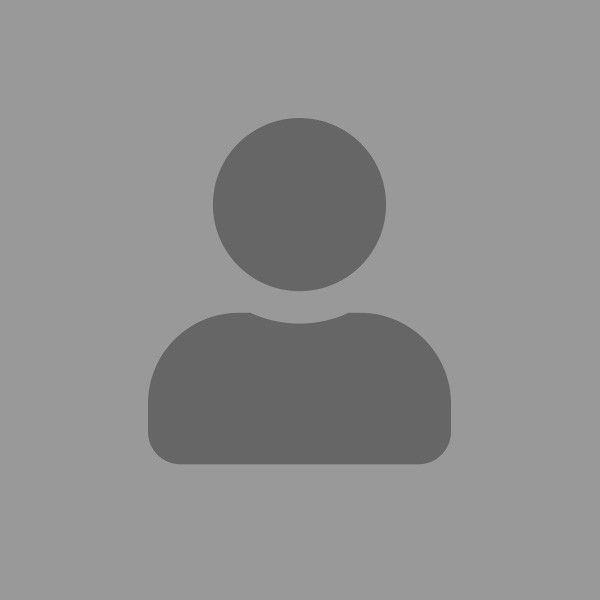
DURHAM, N.C. -- A Duke University Medical Center
neurobiologist has identified key mechanisms by which the
intricate "protein machines" that govern the strength of
connections among neurons build and remodel themselves to
adjust those connections.
Such remodeling of the connections, called synapses, is
central to the establishment of brain pathways during learning
and memory, said the scientists. Also, malfunction of the
synaptic machinery might well play a fundamental role in the
pathology of neurodegenerative disorders including Parkinson's
and Alzheimer's diseases.
The findings were reported in the advanced online version of
the March 2003 Nature
Neuroscience by neurobiologist Michael
Ehlers, M.D.
Said Bill Thies, Ph.D., vice president, medical and
scientific affairs of the Alzheimer's Association, a sponsor of
the research "The discovery of the earliest events in
Alzheimer's disease is very important to understanding the
disease. This paper on activity-dependent synaptic organization
and disorganization opens an interesting path to the earliest
perturbations of Alzheimer's."
The work was also supported by the National Institutes of Health and
other private foundations.
In the Nature
Neuroscience paper, Ehlers reported extensive experiments
revealing the function of a structure known as the
"post-synaptic density" (PSD). The PSD is so named because it
is a thickening of the membrane at the connection point between
neurons, where one neuron receives biochemical signals called
neurotransmitters from its neighbor. Such neurotransmitters are
the means by which one neuron triggers the receiving neuron to
launch a nerve impulse.
"The post-synaptic density has been known for decades as a
distinctive structure readily visible under an electron
microscope," said Ehlers, who is an assistant professor of
neurobiology. "Also, many of its protein components have been
identified -- including neurotransmitter receptors, scaffolding
proteins, signaling enzymes and adhesion molecules. So, it was
clear that this was an important specialized machine for
receiving the chemical signal from the pre-synaptic nerve
cell."
Also, said Ehlers, experiments by other researchers had
shown that the PSD significantly alters its shape in response
to the kind of neural activity that takes place during
learning. They had also established that the neurotransmitter
receptors in the PSD move in and out of the membrane during
such remodeling.
"But what past work has not shown is how the many components
of this machine behave together," said Ehlers. "Our goal was to
take a step back and look at patterns of protein accumulation
and loss, rather than examining one molecule at a time -- to
provide a molecular fingerprint if you will." The key to
achieving such broad insights into PSD remodeling, said Ehlers,
was to explore the gain or loss of a multitude of known PSD
proteins, and not just one or two. Thus, in his experiments,
Ehlers developed a "protein expression profiling" technique to
isolate and measure the levels of some 30 proteins in the PSDs
of cultured rat embryo neurons. This mass analysis revealed a
distinct pattern of protein turnover, he said.
"We found that a significant percentage of the major PSD
protein components moved up and down with neural activity," he
said. "And surprisingly, they didn't behave independently, but
moved as groups or ensembles, with a whole set going up or down
in response to activity. Even more notable was that we saw the
exact mirror image pattern in behavior when neural activity was
blocked." According to Ehlers, such a discovery could have a
profound impact on scientists' ability to understand the
structure and function of the intricately complex protein
machine that is the PSD.
"These findings allow us to begin making testable
predictions about the functional networks of proteins in these
synaptic complexes," he said. "For example, we believe that
there are probably some master organizers in the PSD that
recruit or organize large subsets of these proteins. Now, we
can search for those master molecules." Further, "by providing
a molecular fingerprint of the functional state of the synapse,
we can now begin to compare patterns as the brain develops,
ages, and learns, as well as in disease states such as
Alzheimer's disease, or even across individuals with different
experiences and environmental exposures."
Another surprise, Ehlers found, was the mechanism behind the
turnover of such proteins. In his experiments, Ehlers explored
whether the cell's protein "garbage collection" system might be
involved in the turnover. In this system, proteins targeted for
destruction are tagged with a molecule called ubiquitin and
transported to a shredding complex called the proteasome for
destruction. Ehlers' studies revealed that this system was
required for normal turnover of PSD proteins. Ehlers also
showed that the ubiquitin-proteasome system affected specific
metabolic pathways in the neurons that are known to be involved
in the changes in synaptic connections associated with learning
and memory.
"The prevailing model for long-term plastic change at
synapses has been that genes are switched on to make new
proteins and incorporate them into the synapse," said Ehlers.
"Much, much less appreciated has been the fact that proteins
must also be removed from the structure. And what we found was
that this highly regulated removal is a key part of the
remodeling of the PSD." Also startling, said Ehlers, was the
high base level of remodeling of the PSD.
"I found that neurons in these cultures replace the content
of this signaling machine multiple times a day," said Ehlers.
"And if this recapitulates what's going in the mammalian brain,
this means that synapses are completely turning over all of
their constituents multiple times a day – a stunning finding."
Neuroscientists have long been intrigued in how the brain
changes with learning and experience, a phenomenon called
plasticity. Yet, as Ehlers points out, "perhaps we need to
think more closely about how connections in the brain remain
stable in the face of such incredible ongoing turnover."
"In fact, when I was doing these experiments, I anticipated
a turnover on the order of days, so I took my first time
measurement at about a day and found no protein label left in
the sample. I thought the experiment had failed until I decided
to do measurements at earlier time points."
According to Ehlers, the new insights into the PSD his
studies allow could have important implications for
understanding neurodegenerative diseases including Parkinson's
and Alzheimer's diseases.
"Many of these diseases have as their hallmark pathology
abnormal deposits in the brain, many of which show high levels
of ubiquitin," he said. "For example, there are rare familial
versions of Parkinson's that arises from mutations in genes
that regulate the attachment of ubiquitin to proteins. So,
these findings might give new insights into how such mutations
affect the brain. Such findings might also shed light in the
subtle pathological changes in synaptic connections that
eventually give rise to Alzheimer's disease, he said.
"There is an increasing appreciation of the idea that before
the neurological damage from Alzheimer's disease becomes
apparent, there may be subtle synaptic defects that cause only
a mild cognitive decline," said Ehlers. "This study showing the
central relationship between the ubiquitin system and synaptic
organization gives us a research pathway to trace the possible
origin of these subtle defects. And it is my hope that such
basic insights will lead to therapies to remedy the defects
early."